Introduction:
Recently, the research group led by Professor Min Qiu at Westlake University has successfully developed a novel silicon carbide photonic device that effectively mitigates thermal drift issues in high-power laser processing. Utilizing semiconductor fabrication method, the team fabricated a large-scale, high-precision 4H-SiC metalens that benchmarks against commercial objectives lens and achieves diffraction-limited focusing. After prolonged high-power laser irradiation, the device's performance remained stable, almost unaffected by heat absorption. This achievement represents a significant breakthrough in high-power laser systems, opening new horizons for their application and efficiency enhancement. The related research results were published in the international journal Advanced Materials under the title "4H-SiC Metalens: Mitigating Thermal Drift Effect in High-Power Laser Irradiation."
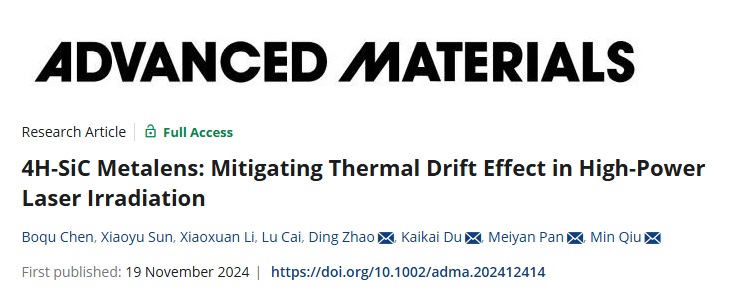
Research Background:
Laser processing is widely utilized in cutting, welding, medical applications, communications, sensing, and military applications. These applications rely on optical components, especially lenses that can withstand high-power lasers and precisely focus laser beams onto specific targets. In conventional laser processing systems, objective lenses are typically composed of cascaded fluorite lenses or synthetic material lenses and are protected by noble gases. However, the low thermal conductivity of lens materials and filling gases poses thermal management challenges: under high-power conditions, significant heat absorption due to Ohmic losses is difficult to dissipate effectively. Consequently, during continuous processing, lenses may deform due to thermal stress or melt, leading to degraded optical performance or even irreversible damage. To address this issue, conventional laser processing systems employ bulky and complex objective lens cooling rings, highlighting the urgent need for compact and efficient alternative solutions.
In recent years, metasurfaces have garnered widespread attention as a promising method for thermal modulation. According to different modulation mechanisms, they can be divided into radiative thermal modulation and conductive thermal modulation. Radiative thermal modulation utilizes structures such as gratings, multilayer films, and photonic crystals to regulate a device's thermal emissivity, but limitations like small temperature modulation ranges and slow response speeds restrict their applications. Conductive thermal modulation is further divided into active and passive control. Active conductive thermal modulation uses external drivers to transfer heat actively, achieving programmable thermal functions on adaptive metasurface platforms, but their bulky size limits integration capabilities. In contrast, passive conductive thermal modulation employs materials with excellent thermal conductivity for thermal modulation, providing fast, stable, reliable, and maintenance-free thermal management. Given the stringent requirements of industrial laser processing on the optical performance of transmissive focusing devices, stability under extreme conditions, device integration, and thermal management capabilities, developing a high-performance, low-thermal-drift metalens with a high laser damage threshold is particularly necessary.
Research Highlights:
In this study, Professor Min Qiu's research group designed and fabricated a monolithic 4H-SiC metalens that achieves optical performance comparable to commercial objectives lens while successfully mitigating thermal drift effects under high-power laser irradiation (as shown in Figure 1). The selected 4H-SiC material offers high refractive index, low loss from visible to near-infrared wavelength, excellent mechanical hardness, chemical resistance, and high thermal conductivity. Optical tests showed that the 4H-SiC metalens possesses optical performance equivalent to commercial objectives. In high-power laser irradiation tests simulating long-term continuous processing under harsh conditions, the 4H-SiC metalens demonstrated stable performance while eliminating dependence on complex cooling systems, opening new application prospects for SiC photonics.
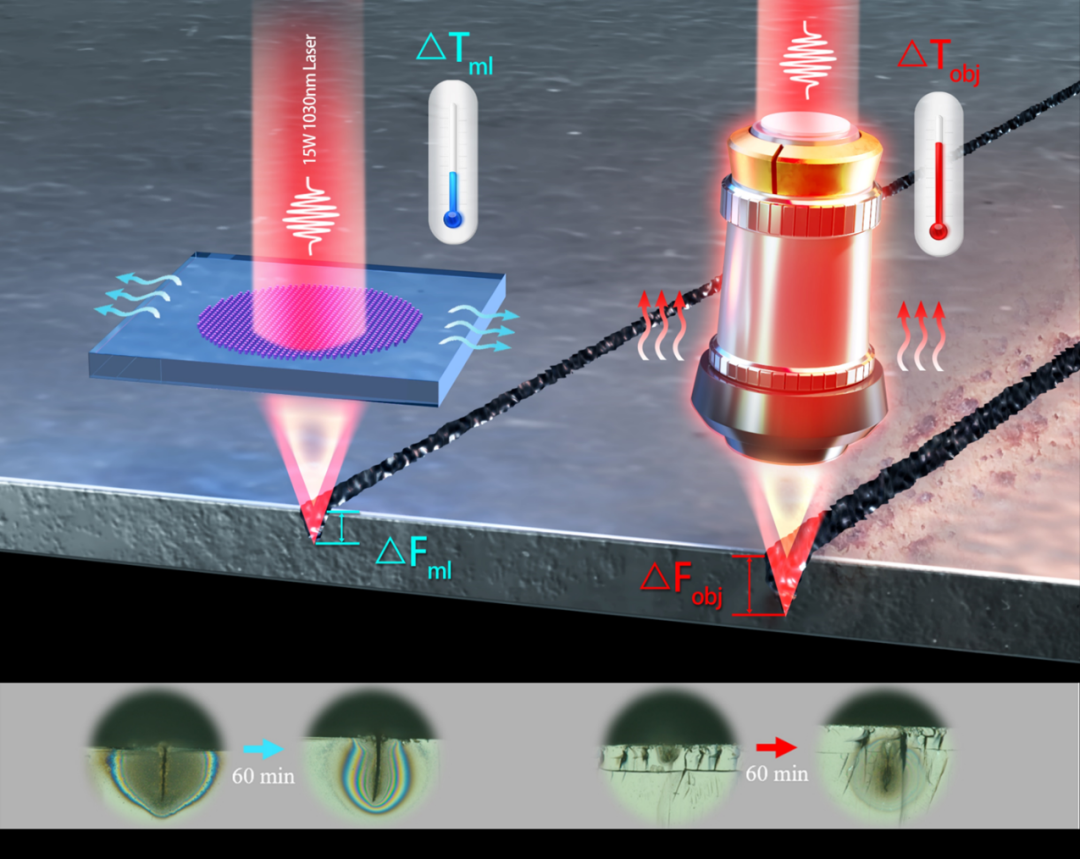
Figure 1: Schematic illustration of the thermal drift effect of the 4H-SiC metalens (left) and traditional objective lens (right)
This 4H-SiC metalens benchmarks against high-performance commercial objectives (Mitutoyo 378-822-5), with design targets of a numerical aperture (NA) of 0.5 and a focal length of 1 cm. Notably, the aperture width of the 4H-SiC metalens is 1.15 cm, larger than the beam size typically produced by high-power lasers, enabling broad adaptability. To balance design and fabrication, the device uses isotropic nanopillars as meta-atoms (as shown in Figure 2a), with a height of H=1 μm, providing dynamic phase as truncated waveguides. The period between adjacent meta-atoms is P=0.6 μm, which can achieve diffraction-limited focusing at this period. Since the birefringence of 4H-SiC can cause slight phase differences between x and y polarized incidence, each meta-atom is optimized by minimizing the quality factor. Ultimately, eight sizes of meta-atoms were obtained (Figures 2b–d), each achieving the corresponding target phase modulation at a wavelength of 1.060 µm, while possessing high transmittance greater than 0.85 and being polarization-insensitive.
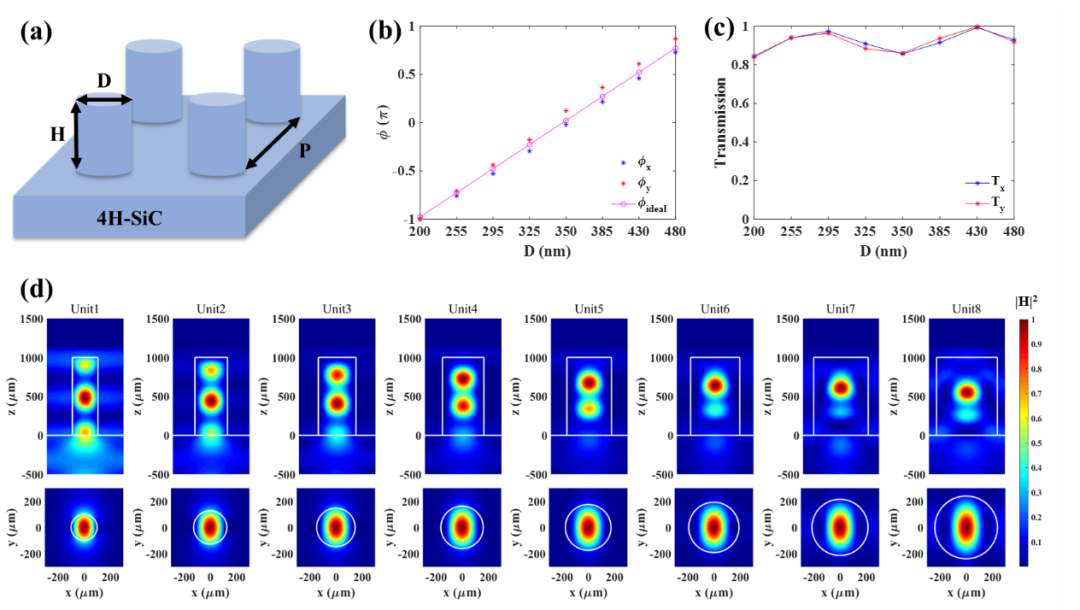
Figure 2: Optical response of 4H-SiC metasurface units
The fabrication of the 4H-SiC metalens employed a series of semiconductor processing techniques, including electron beam lithography, physical vapor deposition, and inductively coupled plasma etching, processing fully filled high-aspect-ratio nanopillars on a substrate surface of 1.15×1.15 cm2. As shown in Figures 3a–e, scanning electron microscopy and atomic force microscopy measurements determined that the structure has a period of 600 nm, a fill factor ranging from 0.3 to 0.78, and a height of 1.009 µm. The sample characterization results demonstrate the excellence of the processing technology, and this large-area, high-precision, high-aspect-ratio metasurface fabrication method can be applied to similar devices, achieving mass production.
The optical performance of the 4H-SiC metalens was tested using a self-constructed transmission microscopic imaging system (as shown in Figure 3f). The system vertically directs a parallel laser with a wavelength of 1030 nm onto the 4H-SiC metalens and achieves CCD imaging through a coaxial microscope system. By performing step-scanning tests within a range of ±35 µm around the focal plane, images of the focal plane and focal field were obtained (as shown in Figures 3g–h). Data analysis showed that the focal field at a focal length of 1 cm presents a smooth Gaussian distribution. The light intensity distribution in the focal plane test exhibited excellent focusing performance (Figures 3i–j), with a full width at half maximum (FWHM) of 2.9 µm. Based on the test results, the focusing efficiency of the 4H-SiC metalens was calculated to be 96.31%. Using an optical power meter to measure the incident and exit surfaces of the 4H-SiC metalens, the transmittance of the device was measured to be 0.71. These optical test results indicate that the 4H-SiC metalens exhibits optical characteristics comparable to commercial objectives and can achieve the same processing capabilities in laser processing systems.
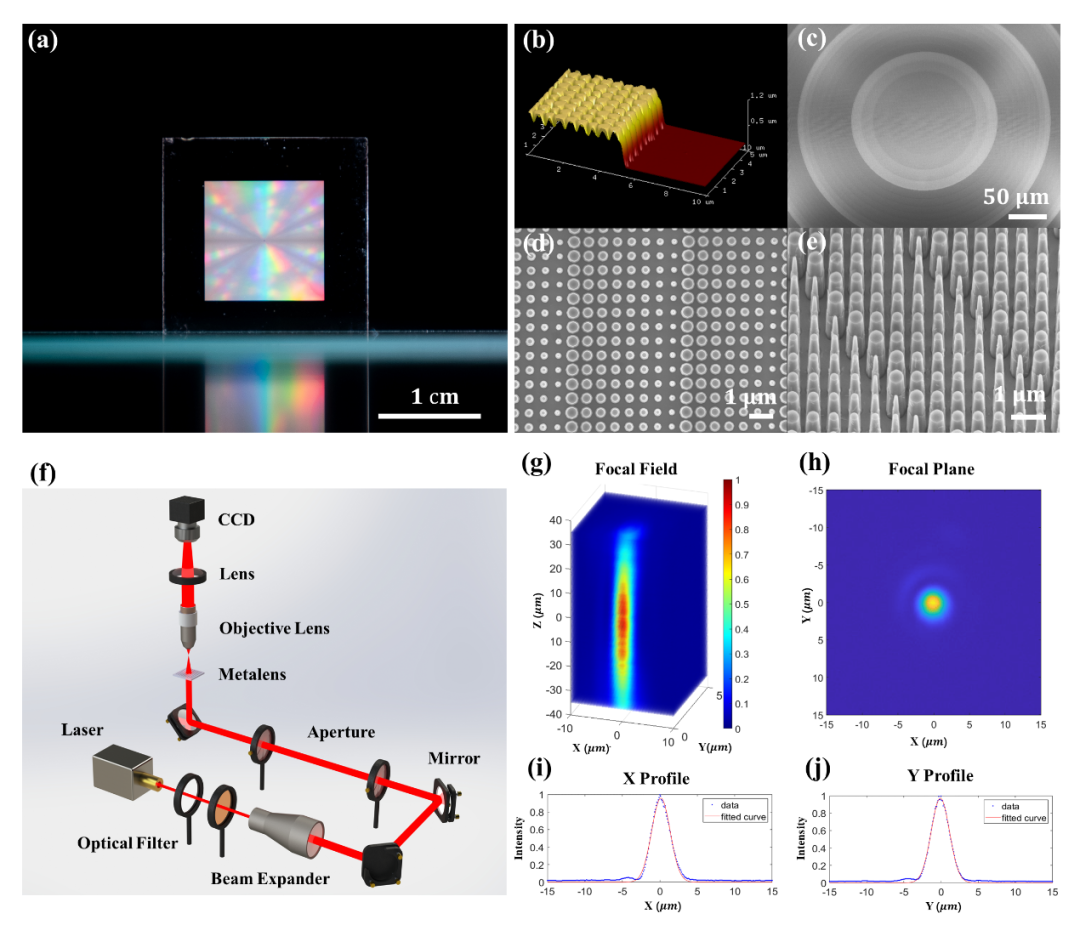
Figure 3: Morphological characterization and optical testing of the 4H-SiC metalens
To simulate harsh high-power continuous processing conditions in laser applications, the thermal drift tests adopted the same optical path as the optical tests, but the light source was replaced with a 15 W 1030 nm laser. The changes in device temperature, focal plane, and cutting effect of the 4H-SiC metalens and the commercial objective lens were separately tested over 1 hour of continuous operation. The device surface temperature changes measured by an infrared thermal imager are shown in Figures 4a–b. After 60 minutes of high-power laser irradiation, the device temperature of the 4H-SiC metalens increased by only 3.2 ℃, which is merely 6% of the temperature change of the objective lens (which increased by 54.0 ℃). Compared to traditional objective lenses, the 4H-SiC metalens reaches a stable temperature after approximately 10 minutes of operation without additional cooling components, displaying smaller temperature changes and lower operating temperatures. This excellent thermal management performance demonstrates the effectiveness of the 4H-SiC metalens under harsh conditions.
To find the changes in the device's optical performance, the focal plane shift over 1 hour was recorded using a CCD (as shown in Figures 4c–d). The test results showed that the focus of the 4H-SiC metalens did not exhibit significant shift, whereas the focus of the commercial objective lens showed noticeable shift after 30 minutes, eventually causing the CCD to be unable to form an image due to excessive displacement. By processing the images to obtain the FWHM and central coordinates of the focal spot and comparing these with the initial position, in-plane displacement data were obtained. After 1 hour of continuous high-power laser irradiation, the displacement of the z-axis stage required to return to the focal plane was recorded, yielding the device's offset along the optical axis. The focal plane shift of the commercial objective lens was 213 µm, while that of the 4H-SiC metalens was only 13 µm, indicating its excellent optical stability and consistency during continuous high-power laser irradiation.
Using the same optical setup, laser cutting experiments were conducted to compare the impact of thermal drift on processing outcomes during actual laser cutting processes. The experiment selected 4H-SiC wafers, which are extremely challenging to cut, as the material. After calibrating the cutting path via step-scan parameter tests, cuts were made along the x-axis every 10 minutes, recording the cutting effects over 1 hour. The cutting morphology of the cross-section of the processed wafers was characterized using an optical microscope (as shown in Figures 4e–f). Results showed that after 60 minutes of operation, the 4H-SiC metalens maintained stable laser cutting performance, while the focus of the commercial objective lens significantly shifted into the substrate after 30 minutes. Data analysis found that the variation in cutting depth of the 4H-SiC metalens after 1 hour was only 11.4% of that of the commercial objective lens. The experimental results validated the focal plane shift tests, demonstrating the superior device stability of the 4H-SiC metalens in practical industrial applications.

Figure 4: Thermal drift tests of the 4H-SiC metalens under high-power laser irradiation
Summary:
This study presents a 4H-SiC metalens that effectively mitigates thermal drift issues in high-power laser processing. Experiments demonstrate that, leveraging its excellent thermal conductivity, the 4H-SiC metalens achieves superior thermal stability and optical performance. The metalens matches the optical specifications of high-performance commercial objectives and achieves polarization-insensitive and efficient focusing based on nanopillar meta-atoms. By employing semiconductor processing techniques compatible with mass production, the fabrication challenges of large-aperture 4H-SiC metalenses were successfully overcome. Experimental results show that the metalens achieves diffraction-limited focusing at the designed focal length and exhibits exceptional stability under continuous high-power laser irradiation, with minimal focal shift—far superior to commercial objective lenses. In laser cutting applications, the variation in cutting morphology using this metalens was smaller. These results highlight the superior performance of the 4H-SiC metalens compared to traditional objectives, which usually require complex cooling systems to achieve similar stability levels.
In the future, with further research and optimization, the 4H-SiC metalens is expected to be widely utilized in high-power laser systems, promoting advances in related fields. With its compact design and excellent optical and thermal performance, this new generation of metasurface devices can be applied in areas such as augmented reality, aerospace, and laser processing, effectively addressing critical thermal management issues in current industries.
Doctoral students Boqu Chen and Xiaoyu Sun, jointly trained by Zhejiang University and Westlake University, are co-first authors of the paper. Professor Min Qiu of Westlake University, Associate Researcher Meiyan Pan of the Jihua Laboratory, Dr. Kaikai Du of Moldnano (Hangzhou) Technology Co., Ltd., and Researcher Ding Zhao of Westlake Institute for Optoelectronics are co-corresponding authors. The research work was supported by the National Natural Science Foundation of China and the Guangdong Basic and Applied Basic Research Foundation projects.
This article is reprinted from: The official public account of the School of Engineering (SOE) at Westlake University.